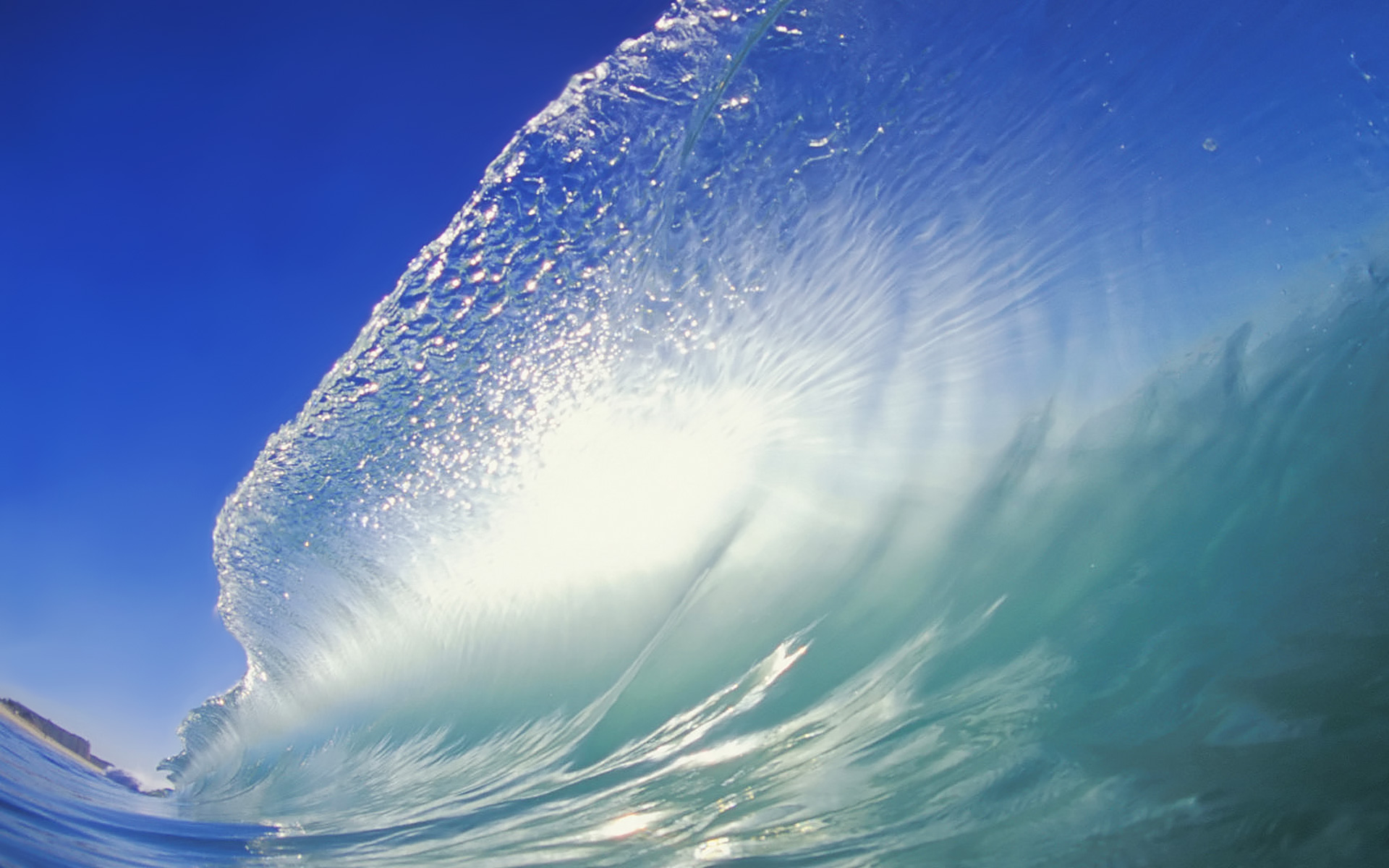
Salinity refers to the dissolved salt content of a body of water. Marine salinity levels are influenced by a number of factors including rainfall, evaporation, inflow of river water, wind, and melting of glaciers. Salinity can have a great impact on the type of organisms that live in a body of water, as examined in the Case Studies section below. Additionally, salinity plays a critical role in the water cycle and ocean circulation. The exact influence of salinity on these two phenomena is explained in this video by NASA. Scientists use robotic floating devices that collect information about ocean salinity and temperature in order to study the water cycle. A recent study by Durack et al. on the intensification of the water cycle as a result of ocean salinity changes in the past fifty years shows that the water cycle has since intensified by 4%, twice the rate predicted by models. Furthermore, the study predicts that a 2°C increase in global temperature would result in a 16% intensification of the water cycle. Similarly, a 3°C increase would see a 24% intensification. The following figure, which shows surface salinity changes from 1950 to 2000, was published along with the study. Regions becoming saltier are depicted in red while blue represents regions becoming fresher.
A Change in the Freshwater Balance of the Atlantic Ocean over the Past Four Decades
The
salinity of oceans, particularly the Atlantic, has changed over the past four
decades. When researchers measured the change in salinity levels of a “long
transect (50° S
to 60° N) through the western basins of the Atlantic
Ocean” (Curry, 2003). They found that the oceans freshened in areas north of 40° N
and south of 25° S. The region between 35° N and 25° S, on the other hand,
experienced an increase in salinity. The freshening of the ocean can be
attributed to “enhanced wind-driven exports of ice or fresh water from the
Arctic, increased net precipitation rates, and/or elevated volumes of continental
runoff from melting ice” (Curry, 2003). Conversely, the increase of salinity levels could be
the result of altered circulation between surrounding waters, intensified trade
winds and/or higher evaporation rates due to warmer ocean surfaces. Salt cannot
be gained nor lost through the atmosphere, so an increase in salinity can only
occur via (1) a transfer of additional salt from surrounding waters or (2) the
removal of fresh water. Because surrounding waters do not exhibit the
corresponding decrease in salinity, the increase in salinity levels must be due
to higher evaporation rates.
The Impact of Climate Change on the Adaptation of Fish in the Baltic Sea
Climate change has influenced the development and evolution of marine species in the Baltic Sea since the last glaciation. The distribution and abundance of marine fish in this area is heavily dependent on the salinity, temperature, and oxygen content of the different water layers. The salinity and oxygen content of the Baltic Sea are determined by the intensity of water exchange between it and the North Sea. Water exchange, in turn, is controlled by meteorological processes which could change along with the climate. Because salinity and oxygen content can vary significantly from year to year, successful establishment of the Baltic Sea by marine species is difficult. For instance, “of about 120 marine fish species in the North Sea, fewer than 30 occur permanently and can reproduce in [the Baltic Sea]” (Ojaveer, 2005). These numbers are quiet alarming since they show that less than one quarter of marine species in the vicinity of the Baltic Sea have been able to adapt to the salinity levels which are constantly changing as a result of climate change. The adaptations employed by the few marine species that have been able to establish themselves in the Baltic Sea are very interesting. Species with floating eggs have altered their specific gravity/egg diameter ratios effectively changing the density of their eggs. The spawning period of some species in the Baltic Sea starts earlier and lasts longer than that of their counterparts in the west. Additionally, these species mature faster and exhibit higher reproductive ability. The few marine species that have been able to adapt may soon see those adaptations become obsolete as the salinity levels change beyond the normal year-to-year range. This is a great case study of how climate change may semi-directly affect marine species in the Baltic Sea.
Effects of Salinity on Oxygen Consumption of Cyprinodon Variegatus
The
sheepshead minnow (Cyprinodon varuegatus) is euryhaline, meaning it is
able to adapt to a wide range of salinities. The minnows are commonly found in the
shoreline (1-2 cm deep) of saline lakes in San Salvador Island. Freshwater
influx and evaporation can cause changes in the salinity of the lakes. In
particular, heavy rains can quickly dilute the shallow waters. Researchers
studied the extent to which changes in osmotic regulation (as a result of
salinity differences) are accommodated by shifts in metabolic rates (measured
by changes in oxygen consumption). The experiment was carried out by placing
the minnows in water of varying salinity (10% and 35%) and measuring changes in
dissolved oxygen content to determine the amount of oxygen consumed. Results
showed that oxygen consumption was greatest among the minnows exposed to the
dilute (10%) environment. Researchers concluded that reduced salinities are
associated with increased metabolic rates, as these elevated rates are required
for osmotic adjustment. This study is of importance as it quantifiably demonstrates
how changes in salinity can affect the metabolism of marine organisms such as
minnows.
Effect of Salinity on Cadmium Uptake by the Tissues of the Shore Crab Carcinus Maenas
Cadmium is a naturally occurring element which is present in
ocean water in trace quantities. Although cadmium does not have any physiological
functions, many marine organisms are known to accumulate the element in their
bodies. The rate of accumulation is affected by both temperature and salinity. D.
A. Wright used Carcinus maenas, a
euryhaline shore crab, to study the effects of salinity on the accumulation of
cadmium by marine organisms. The experiment consisted of exposing the specimens
to two solutions of differing salinity and measuring their cadmium uptake
throughout a 68-day period. Wright examined cadmium concentration in tissue
samples taken from the carapace, hepatopancreas, gills, and chelae muscle. According
to the data, salinity had no effect on the cadmium concentration of the
hepatopancreas or the chelae muscle. However, cadmium accumulation in the
carapace and gills was significantly higher in dilute sea water. These results
are consistent with those of other studies. Similar studies conducted on Mytilus edulis (blue mussel) and Artemia franciscana (brine shrimp) also
show an increase in cadmium accumulation at lower salinities. Additionally, a
study published by David Engel in an issue of Environmental Health Perspectives affirms that
decreased cadmium toxicity is observed at higher salinities. While these
studies may not address climate change directly, their findings can be combined
with the ideas discussed in the previous case studies to formulate some predictions.
The study by Ruth Curry showed that certain bodies of water are becoming less
saline as a result of increased runoff from melting ice. The present case study
showed that decreased salinity leads to increased cadmium accumulation across a
wide array of marine organisms. Humans likely consume some of the marine organisms
whose cadmium uptake is influenced by ocean salinity. Therefore, one can
predict that humans may become exposed to more cadmium as bodies of water
become more dilute. This prediction is terrifying considering cadmium is toxic
and may cause kidney and developmental disorders.
Effect of Salinity on Freshwater Ecosystems in Australia
Under normal circumstances, salt enters bodies of water either
through groundwater or the atmosphere, the latter occurring simply when salt is
transported by wind and rain. The groundwater route involves the erosion and
weathering of soils and rocks as underground water flows. As underground water
levels rise, more salt is dissolved and deposited in nearby bodies of water.
Large scale clearing of native vegetation is one reason why the underground
water levels may rise. Native vegetation would normally trap water before it
made its way to the underground watertable. The lack of native vegetation,
often replaced by pastures, increases the amount of water that reaches the
watertables causing underground water levels to rise and salinity to increase. Changes
in salinity levels affect not only marine life but also the physical component
of aquatic ecosystems. From a physical environment standpoint, increased
salinity levels may help remove particles from the water column, which in turn
increases water clarity. Clearer water means more light penetration and
therefore higher rates of photosynthesis. Increases in photosynthesis may cause
blooms of cyanobacteria which could alter dissolved oxygen levels. Changes in
salinity levels can also affect the abundance and solubility of some minerals,
particularly the major ionic species. The ionic composition of water “modifies
the way biota respond to high salinities,” and may also have a slight effect on
water acidity (Nielsen et al., 2003). As
for marine life, increased salinity levels may slow organismal growth and
reduce species richness. Models predict the Australian freshwater rivers and
wetlands will experience salinity levels ranging from 500 mg L-1 to
above 10,000 mg L-1 over the
next 50 years. These increased salinity levels affect each form of life differently.
Algae, for example, cannot tolerate salinity levels above 10,000 mg L-1 which
means diversity would be severely decreased if salinity levels reach this
level. Plants, on the other hand, can only tolerate salinity levels up to 1,000
mg L-1 before their growth and reproduction is impaired.
At levels above 4,000 mg L-1 , halophytic species replace all
non-halophytic plant species, reducing diversity. Invertebrates are also
affected by increased salinity levels, displaying a rapid decrease in diversity
when salinity levels exceed 1,000 mg L-1 but a less rapid decline at
levels above 10,000 mg L-1. Lastly, most Australian fish appear to
be tolerant of salinity levels well above 3,000 mg L-1.
Unfortunately, salinity levels above 3,000 mg L-1 do affect juveniles and eggs, often
reducing survival rate by 50%. Although the study by Nielsen has some upsetting results, the findings can help set salinity level limits that could give conservation agencies a more quantitative value to work with in their efforts to save Australian marine life.
Barton, M. "Effects of Salinity on Oxygen Consumption of Cyprinodon variegatus." Copeia , Vol. 1987, No. 1 (1987), 230-232. Web
Curry, R., B. Dickson, and I. Yashayaev. "A Change in the Freshwater Balance of the Atlantic Ocean over the past Four Decades." Nature 426.6968 (2003): 826-29. Web.
Ojaveer, E., and M. Kalejs. "The Impact of Climate Change on the Adaptation of Marine Fish in the Baltic Sea." ICES Journal of Marine Science 62.7 (2005): 492-500. Web.
Back to Introduction
Comments
kevini
Nov 28, 2012
Props to Ikcrites and Fawcette for adding pictures. Yay!